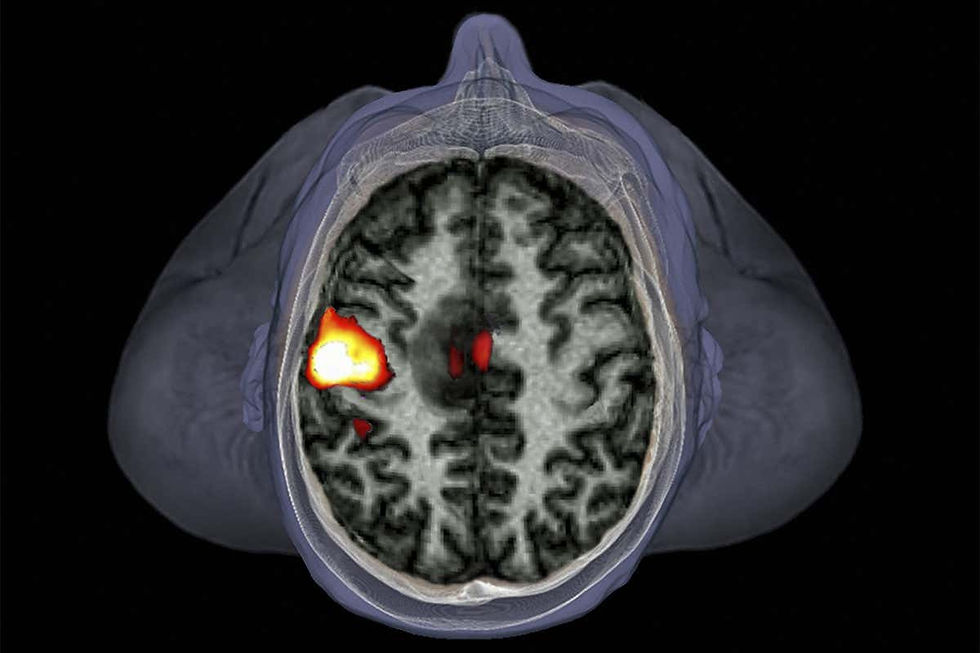
Epilepsy is a network disease that cannot be reduced solely to the dysfunction of the seizure-onset zone (Shamshiri et al., 2019). This network must be adequately disrupted to prevent future seizures (Luders et al., 1993). Considering epilepsy is prevalent in ~1% of the population, with 30% of cases being intractable to anti-epileptic drugs (AEDS), surgical interventions to resect the epileptogenic zone is a desirable option (Pittau et al., 2014; Maguire et al., 2020). The epileptogenic zone is “the area of cortex that is necessary and sufficient for initiating seizures and whose removal (or disconnection) is necessary for complete abolition of seizures” (Luders et al., 1993). Theoretically, post-operative seizures will continue if part of the epileptogenic zone remains intact (Jehi, 2018). Therefore, the surgeon should target the epileptogenic zone while preserving eloquent cortex responsible for language (Rosenow & Luders, 2001).
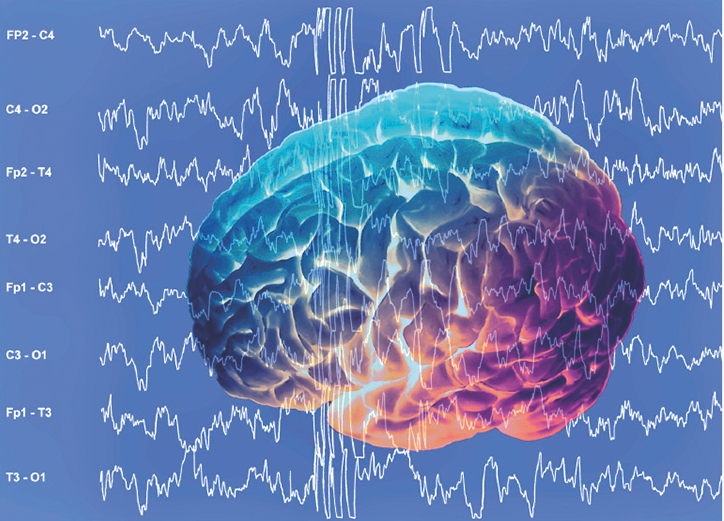
The epileptogenic zone cannot be measured directly; rather, its location is inferred indirectly after defining the irritative zone, seizure-onset zone, symptomatogenic zone, epileptogenic lesion, and functional deficit zone (Rosenow & Luders, 2001). The delineation between the cortical zones of an epilepsy network and language mapping requires multimodal techniques for surgical planning. Although intracranial electroencephalography (EEG) eliminates the distance of the recording electrode and insulating barriers, it is costly and risky with potential complications including bleeding and infection (Zhang et al., 2014). Scalp EEG and functional magnetic resonance imaging (fMRI) are the preferred non-invasive techniques to identify these zones (Mele et al., 2019; Abreu et al., 2018). A strength of fMRI, which investigates brain activations during resting state and task executions, is excellent spatial resolution (Mele et al., 2019). However, fMRI suffers from the temporal inverse problem, meaning brain maps showing regional activations do not allow for inferences about “when” they occurred (Huster et al., 2012). On the contrary, EEG uses electrodes to record brainwave activity to the order of milliseconds, providing excellent temporal resolution (Mele et al., 2019). Simultaneous EEG-fMRI acquisition provides data about electrical activity as well as cerebral blood flow as a measure of neural activity and overcomes the limitations of a single modality (Hamandi et al., 2004; Mele et al., 2019; Huster et al., 2012).
Multimodal Acquisition for Surgical Planning
Pre-surgical evaluations aim to localize the epileptogenic zone to guide decisions regarding the resection margin (Ito et al., 2021). An effective epilepsy surgical strategy should aim to reduce symptoms, protect language production/reception, and focus on the concept of quality of life. Surgical planning and outcomes are better for patients that have an epileptogenic lesion (Zhang et al., 2014). A 3T MRI protocol that provides thin cuts through temporal lobes reveals subtle lesions including mesial temporal sclerosis or cortical dysplasia (Wellmer et al., 2013; Duncan et al, 2016; Wehner & Luders, 2008; Coan et al. 2014). EEG-fMRI maps hemodynamic changes related to an interictal epileptiform discharge (IED) (Shamshiri et al., 2019; Zijlmans et al., 2007; Thornton et al., 2011). Upon brain activation of recruited networks, a net increase in blood oxygenation leads to a blood oxygenation level dependent (BOLD) signal increase due to magnetic susceptibility differences induced by varying concentrations of paramagnetic deoxyhemoglobin relative to diamagnetic oxyhemoglobin (Abreu et al., 2018). Removing the max BOLD changes causes 70% seizure freedom whereas resection of the complete BOLD change causes 90.9% seizure freedom a year after epilepsy surgery (Pittau et al., 2014). EEG-fMRI provides valuable diagnostic and prognostic information for determining epileptogenic zone localization that may modify the surgical plan, including in patients that were previously rejected for surgery due to unclear focus or presumed multifocality (Szaflarski et al., 2017; Hamandi et al., 2004; Zijlmans et al., 2007; Zhang et al., 2014; Markoula et al., 2018). The data allows for better targeting of surgical resection and improves the implantation of intracranial electrode arrays if needed (Grouiller et al., 2011).
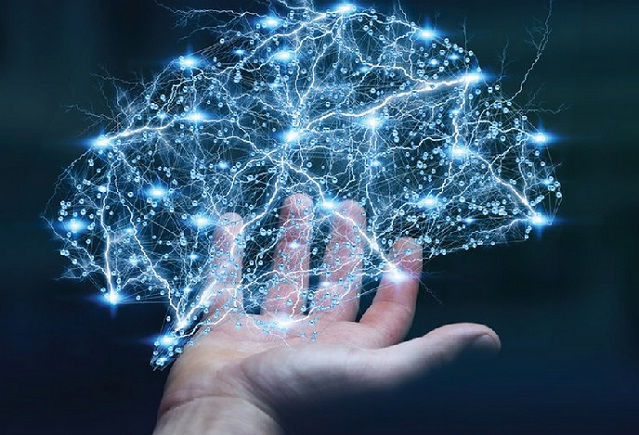
Neural Networks
Epileptic activity disrupts the brain’s intrinsic connectivity networks responsible for awareness, attention, perception, and cognitive control (Shamshiri et al., 2019). At baseline, patients with epilepsy may have decreased executive functioning, deficits in aspects of verbal generative fluency and naming ability, and poor memory encoding due to dysfunctional activity in the epilepsy neural network involving the frontotemporal lobes (Shamshiri et al., 2019; Drane & Pedersen, 2019). Spencer (2002) defines a neural network as “functionally and anatomically connected, bilaterally represented set of cortical and subcortical brain structures and regions in which activity in any one part affects activity in all others.” Any part of the network may produce seizure activity with variable participatory order of involvement within structures (Spencer, 2002). Epilepsy networks can be altered through structural interruptions and modifications of electrical, biochemical, or metabolic activity (Spencer, 2002). These factors influence the frequency and severity of seizures (Spencer, 2002). Neural networks in temporal lobe epilepsy (TLE) patients have increased path lengths and increased local clustering, which creates a more lattice-like configuration related to increased local but decreased global efficiency (Tavakol et al., 2019). Moreover, their neural networks have a high degree of synchronizability and hyperconnectivity (Van Straaten & Stam, 2013; Bonilha et al, 2013; Iannotti et al., 2020).
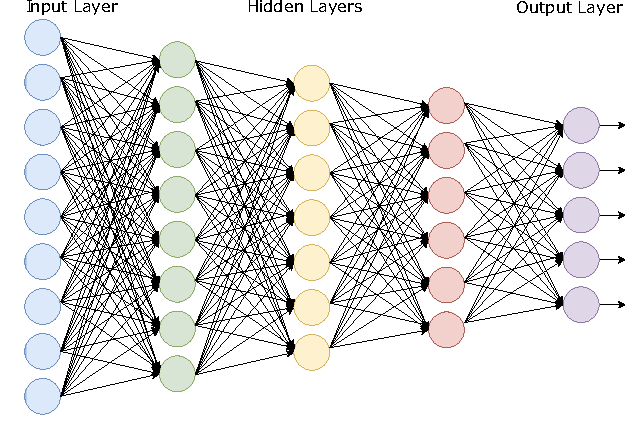
A neural network can be represented mathematically with a graph made up of vertices or nodes for brain regions and edges for connections in terms of functional or structural relationships between nodes (Van Straaten & Stam, 2013). The average human adult brain contains 2 x 10^10 neurons and 15 x 10^13 synapses resulting in a mean number of connections of 7000 for each neuron (Van Straaten & Stam, 2013). Longer connections are older and each edge can carry a value (based on strength, length, or importance) and direction (depending on the influence of one node on another) (Van Straaten & Stam, 2013). Understanding how patients' network metrics deviate from a completely random network (of the same size and mean connectivity) can help predict surgical outcomes (Sinha et al., 2017; Van Straaten & Stam, 2013). After determining the number of abnormal nodes before surgery and the amount that is expected to be spared after surgery, a machine-learning framework can be used to estimate patient-specific chances of post-operative seizures (Ryvlin & Rheims, 2008; Sinha et al., 2021). The pre-surgical findings, including clinical and EEG-fMRI data can help tailor these artificial neural networks localize the seizure focus (Ryvlin & Rheims, 2008; Tavakol et al., 2019).
Interictal Epileptiform Discharges
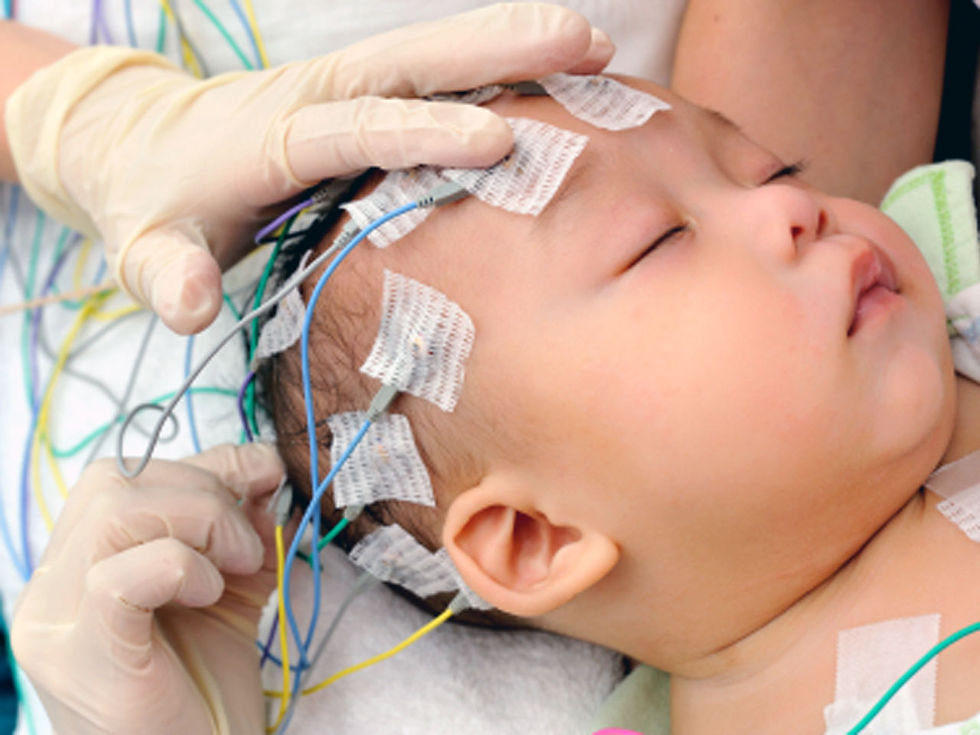
Epileptiform (70ms spikes or 70-200ms sharp waves) that occurs between seizure activity (i.e. interictally) in the irritative zone typically present in short paroxysmal bursts, in a periodic fashion, or as an isolate (Murta et al., 2015; Rosenow & Luders, 2001; Thornton et al., 2011). IEDs are felt to be a marker of poor cognitive prognosis likely due to transient impairments of the neural network (Shamshiri et al., 2019). This disruption is more prominent during task recruitment; for example, the amount of IEDs increased in the temporal lobe during tasks that involved temporal structures (Shamshiri et al., 2019). During EEG-fMRI studies, individuals with left sided IEDs made verbal task errors whereas those with IEDs in the right hemisphere made non-verbal task errors (Shamshiri et al., 2019). Changes in metabolism and blood flow of these brain regions can be examined using fMRI BOLD responses (Sadjadi et al, 2021). Overlapping IED-related BOLD maps with surgical resection has a favourable prognosis in both children and adults (Shamshiri et al., 2019).
Surgical Resection Outcomes
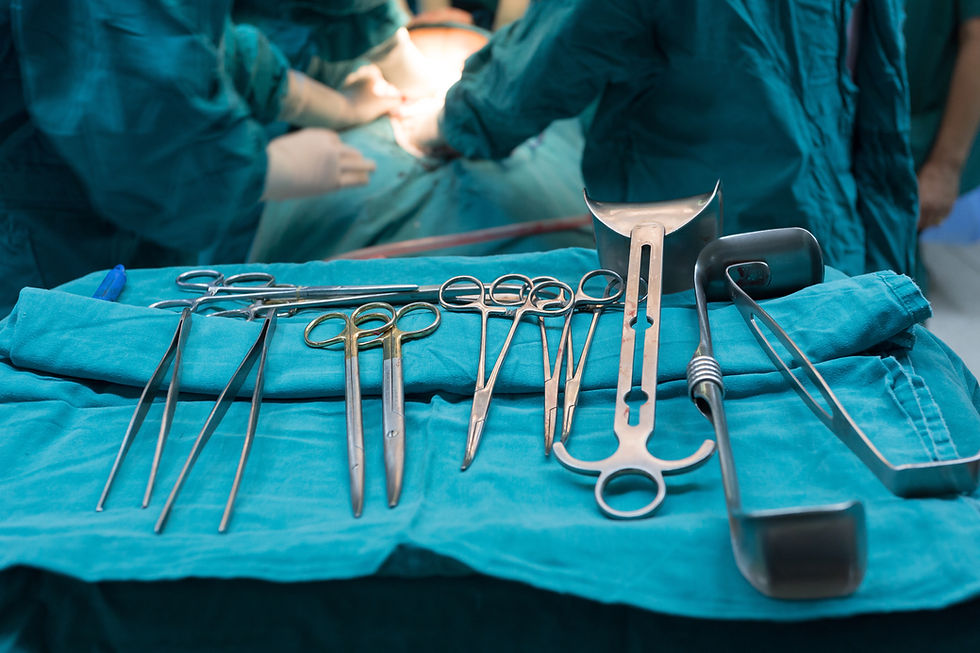
The functional integrity of resected tissues and of remnant brain influence surgical resection outcomes (Vakharia et al., 2018). Some patients with memory deficits at baseline fear that epilepsy surgery will make their memory worse; however, memory might improve due to better seizure control (Engel, 2016). Typically, the memory encoding networks of TLE patients change across both verbal and visual domains, with compensatory engagement of the contralateral hippocampus (Sidhu et al., 2016). Leftward activation asymmetry on fMRI predicts post-operative verbal memory decline (Szaflarski et al., 2017; Sidhu et al., 2015). Comparing health outcomes of patients randomly assigned to a surgical group or medical group (on AEDs for 1 year) revealed that surgery resulted in improved quality of life with better seizure management (Wiebe et al., 2001). The surgical group had 58% seizure freedom compared to 8% in the medical group (Wiebe et al., 2001). Moreover, one of the patients in the medical group died (Wiebe et al., 2001). Some patients experience the “burden of normality” after surgery due to an abrupt change of seizure reduction, which destabilizes relationships predicated on dependency (Vakharia et al., 2018). Most epilepsy surgical patients have substantial and long-lasting decrease in seizure frequency (Mohan et al., 2018). Surgical resections for TLE cause seizure remission up to 80% and long-term complete seizure freedom in 41% (Taylor et al., 2018). Following surgery, many epileptic patients can discontinue AEDs (Friedman, 2014).
Urgency for Epilepsy Evaluations
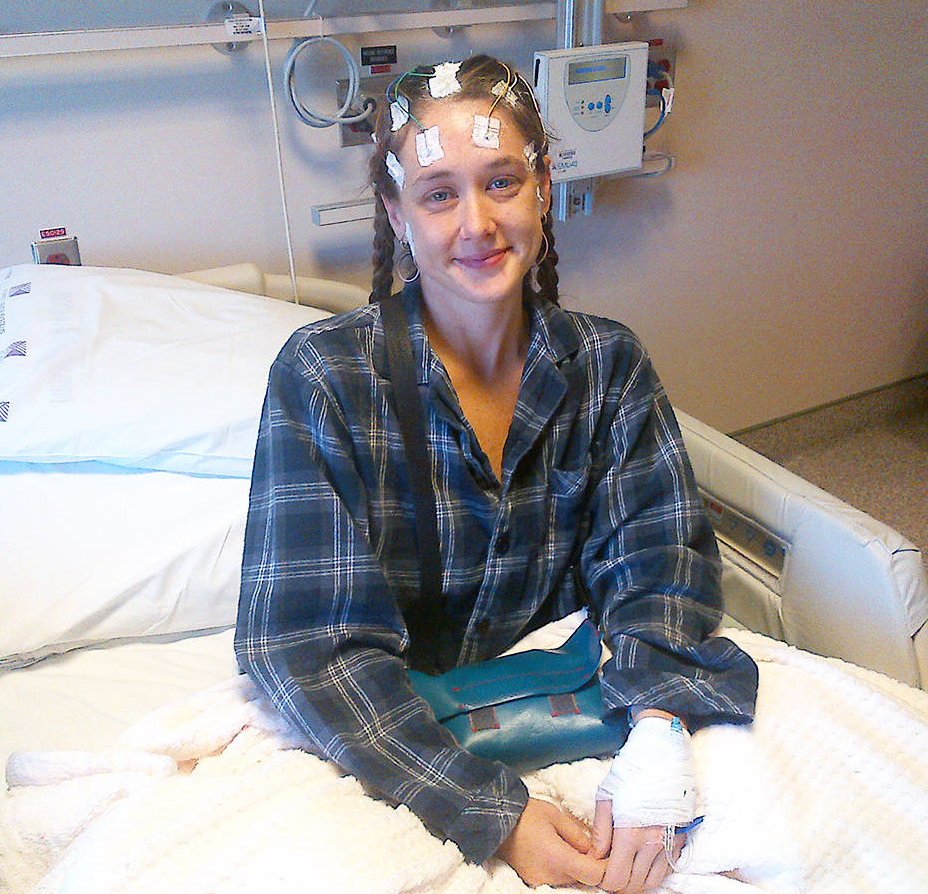
Patients with medication-resistant epilepsy (based on failure of two AEDs due to inefficacy and not tolerance) have a mortality rate that is 5-10 times higher than the general population (Engel, 2016). Sudden Unexpected Death in Epilepsy (SUDEP) does not reveal structural or toxicological reasons for death upon postmortem examination and it occurs in 1% of epileptic patients (Maguire et al., 2020). Presence and frequency of generalized tonic-clonic seizures, male sex, early onset of seizures, duration of epilepsy, and polytherapy are the predictors of SUDEP (Maguire et al., 2020). Continuous seizures increase the risk of SUDEP and decreases quality of life; therefore, early referral for pre-surgical evaluations can help prevent premature death (Engel, 2016; Maguire et al., 2020). In the United States, less than 1% of patients with epilepsy are provided with thorough pre-surgical evaluations with specialized testing at an epilepsy centre (Engel, 2016). These sites also investigate underlying causes, drug choices, drug dosage, and lifestyle issues that may be contributing to seizures (Engel, 2016; Son & Kim, 2016). Numerous investigations help minimize the risk of post-operative neurological, cognitive, or psychiatric deterioration (Ryvlin & Rheims, 2008).
Comparison of Wada Test and fMRI
Dr. Juhn Wada introduced the intracarotid amobarbital procedure (IAP), also known as the Wada test, which is utilized to lateralize language dominance and memory functions of a cerebral hemisphere through temporary inactivation of the opposite hemisphere (Rosenow & Luders, 2001). The eloquent cortex, responsible for language functions, should be avoided during epilepsy surgery to prevent functional deficits (Rosenow & Luders, 2001; Ryvlin & Rheims, 2008). Many researchers argue that fMRI and neurocognitive assessment will replace the Wada test to lateralize language dominance and predict memory capacities before epilepsy surgery (Ryvlin & Rheims, 2008; Benjamin et al., 2018; Szaflarski et al, 2008). One study used fMRI tasks and Wada tests for 38 epileptic patients and 50 healthy controls and found significant correlation for language laterality (Szaflarski et al, 2008). Language dominance using fMRI has been found to be 85% accurate and mirror comparative Wada test data (Benjamin et al., 2017).
Eloquent Cortex
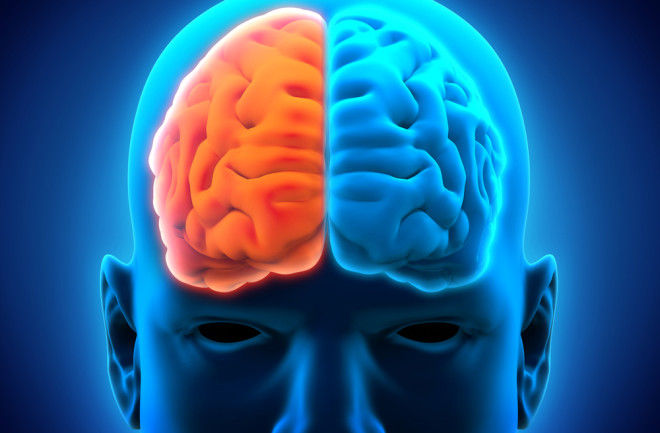
Although most people are left hemisphere dominant for language functions, there is an increased chance of atypical language representation (bilateral or right hemisphere dominant) in those with epilepsy (Drane & Pedersen, 2019). Disruption to the non-dominant temporal lobe impacts the ventral visual processing stream, the “what is it pathway”, thereby causing recognition deficits such as inability to identify famous people or landmarks (Drane & Pedersen, 2019). A dysfunctional dominant temporal lobe does not decrease recognition capability but it does result in significant naming deficits (Drane & Pedersen, 2019).
Pre-surgical fMRI mapping consistently identifies six language areas the surgeon should avoid during resection: Broca’s area (for speech production and articulation), Wernicke’s area (for speech comprehension), supplementary speech area (for sequencing motor movements of speech), basal temporal language area (for word processing and semantic association), Exner’s area (for writing by transforming a word’s phonological representation into a motor command), and angular gyrus (for reading by transitioning between written and spoken language) (Benjamin et al., 2017). These language sites are remarkably similar in terms of topographical regions across individuals, even in children with congenital epilepsy or brain tumours (Drane & Pedersen, 2019). Children do not show evidence of language sites reorganization despite a lesion or seizure focus being in these regions (Drane & Pedersen, 2019).
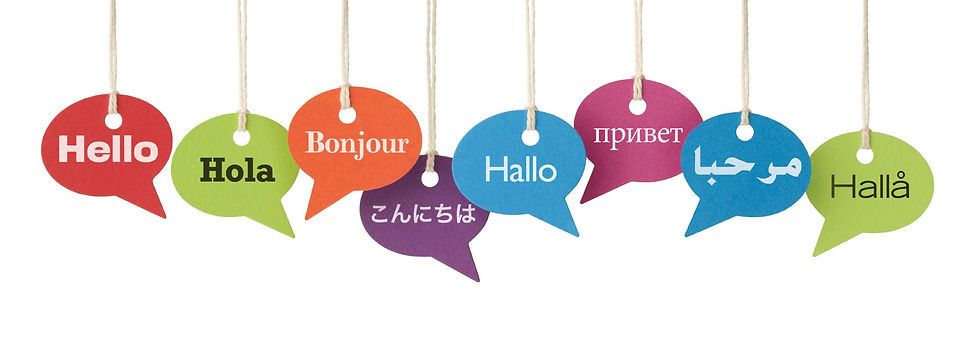
Limitations of EEG-fMRI
The rate-limiting step is post-processing as opposed to EEG-fMRI data acquisition (Huster et al., 2012). The methods in terms of optimal thresholding strategy varied between studies; this impacts analysis decisions and resulting brain maps which is complicated by noise, especially gradient artifact and pulse artifact (Hauf et al., 2012; Benjamin et al., 2017; Abreu et al., 2018). In addition, EEG hardware causes artifacts on the images and improper electrodes can heat via magnetic resonance (MR) radio frequency transmission resulting in discomfort or burns (Mele et al., 2019). Simultaneous EEG-fMRI requires EEG hardware that is safe and compatible within the scanner and MR environment (Mele et al., 2019). EEG-fMRI may have poor yield if there are infrequent epileptiform discharges (Centeno et al., 2016). This can be improved by increasing the scan duration but that increases the likelihood of movement artifact (Centeno et al., 2016). Upon review of the literature, very few studies provided quantitative data about pre-operative seizures and nearly all were retrospective in design (Engel et al., 2003). There is a need for randomized controlled trials as well as larger patient samples in the future to assess the clinical utility of EEG-fMRI in pre-surgical evaluations (Benjamin et al., 2018).
Conclusion
Simultaneous EEG-fMRI is becoming increasingly popular for surgical planning of epileptic patients. This leads to the question, what is the clinical impact of combining EEG and fMRI findings (i.e. IEDs and BOLD response) on assessing the epileptogenic zone in patients requiring surgical intervention? Moreover, how does simultaneous EEG and fMRI data from epilepsy patients' pre-surgical evaluations correlate with seizure reduction post-operatively? Pre-surgical evaluation through combination of fMRI and EEG will improve the accuracy of localizing the epileptogenic zone, reduce surgical risks for the patient and improve seizure outcomes (reduced severity or frequency of seizures). The findings will be used to decide how much brain tissue can be removed safely, particularly without creating deficits in language areas, whilst aiming for seizure freedom post-operatively.
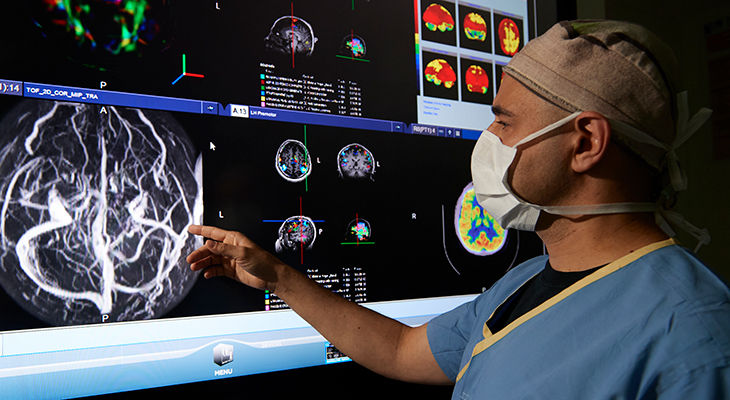
Abreu, R., Leal, A. & Figueiredo, P. (2018). EEG-informed fMRI: A review of data analysis methods.
Frontiers in Human Neuroscience, 12(29). https://doi.org/10.3389/fnhum.2018.00029
Benjamin, C.F.A., Dhingra, I., Li, A.X., Blumenfeld, H., Alkawadri, R., Bickel, S., Helmstaedter, C.,
Meletti, S., Bronen, R.A., Warfield, S.K., Peters, J.M., Reutens, D., Polczynska, M.M., Hirsch, L.J., & Spencer, D.D. (2018). Presurgical language fMRI: Technical practices in epilepsy surgical planning. Human Brain Mapping, 39(10), 4032-4042. https://doi.org/10.1002/hbm.24229
Benjamin, C.F., Walshaw, P.D., Hale, K., Gaillard, W.D., Baxter, L.C., Berl, M.M., Polczynska, M., Noble,
S., Alkawadri, R., Hirsch, L.J., Constable, R.T., & Bookheimer, S.Y. (2017). Presurgical language fMRI: Mapping of six critical regions. Human Brain Mapping, 38(8), 4239-4255.
Bonilha, L., Helpern, J.A., Sainju, R., Nesland, T., Edwards, J.C., Glazier, S.S. & Tabesh, A. (2013).
Presurgical connectome and postsurgical seizure control in temporal lobe epilepsy.
Neurology, 81. https://doi.org/10.1212/01.wnl.0000435306.95271.5f
Centeno, M., Tierney, T.M., Perani, S., Shamshiri, E.A., St.Pier, K., Wilkinson, C., Konn, D., Banks, T.,
Vulliemoz, S., Lemieux, L., Pressier, R.M., Clark, C.A., Cross, J.H. & Carmichael, D.W. (2016).
Optimising EEG-fMRI for localization of focal epilepsy in children. Public Library of Science
One, 11(2). https://doi.org/10.1371/journal.pone.0149048
Coan, A.C., Kubota, B., Bergo, F.P.G., Campos, B.M. & Cendes, F. (2014). 3T MRI quantification of
hippocampal volume and signal in mesial temporal lobe epilepsy improves detection of
hippocampal sclerosis. American Journal of Neuroradiology, 35(1), 77-83.
Drane, D.L. & Pedersen, N. (2019). Knowledge of language function and underlying neural
networks gained from focal seizures and epilepsy surgery. Brain and Language, 189, 20-33.
Duncan, J.S., Winston, G.P., Koepp, M.J., Ourselin, S. (2016). Brain imaging in the assessment for
epilepsy surgery. The Lancet Neurology, 15(4), 420-433. https://doi.org/10.1016/S1474-
Engel, J. (2016). What can we do for people with drug-resistant epilepsy? Neurology, 87(23),
2483-2489. https://doi.org/10.1212/WNL.0000000000003407
Engel, J., Wiebe, S., French, J., Sperling, M., Williamson, P., Spencer, D., Gumnit, R., Zahn, C.,
Westbrook, E. & Enos, B. (2003). Practice parameter: Temporal lobe and localized neocortical resections for epilepsy. Epilepsia, 44(6), 741-751. https://doi.org/10.1046/j.1528-1157.2003.48202.x
Friedman, E. (2014). Epilepsy imaging in adults: Getting it right. American Journal of
Roentgenology, 203(5), 1093-1103. https://doi.org/10.2214/AJR.13.12035
Grouiller, F., Thornton, R.C., Groening, K., Spinelli, L., Duncan, J.S., Schaller, K., Siniatchkin, M.,
Lemieux, L., Seeck, M., Michel, C.M. & Vulliemoz, S. (2011). With or without spikes:
Localization of focal epileptic activity by simultaneous electroencephalography and
functional magnetic resonance imaging. Brain, 134(10), 2867-2886.
Hamandi, K., Salek-Haddadi, A., Fish, D.R., & Lemieux, L. (2004). EEG/functional MRI in epilepsy:
The queen square experience. Journal of Clinical Neurophysiology, 21(4), 241-248.
Hauf, M., Jann, K., Schindler, K., Scheidegger, O., Meyer, K., Rummel, C., Mariani, L., Koenig, T. &
Weist, R. (2012). Localizing seizure-onset zones in presurgical evaluation of drug-resistant
epilepsy by electroencephalography/fMRI: Effectiveness of alternative thresholding
strategies. American Journal of Neuroradiology, 33, 1818-1824.
Huster, R.J., Debener, S., Eichele, T. & Herrmann, C. (2012). Methods for simultaneous EEG-fMRI:
An introductory review. The Journal of Neuroscience, 32(18), 6053-6060.
Iannotti, G.R., Preti, M.G., Grouiller, F., Carboni, M., De Stefano, P., Pittau, F., Momjian, S.,
Carmichael, D., Centeno, M., Seeck, M., Korff, C.M., Schaller, K., Van De Ville, D., Vulliemoz, S.
(2020). Modulation of epileptic networks by transient interictal epileptic activity: A dynamic
approach to simultaneous EEG-fMRI. NeuroImage: Clinical, 28.
Ito, Y., Maesawa, S., Bagarinao, E., Okai, Y., Nakatsubo, D., Yamamoto, H., Kidokoro, H., Usui, N.,
Natsume, J., Hoshiyama, M., Wakabayashi, T., Sobue, G. & Ozaki, N. (2021). Subsecond EEG-
fMRI analysis for presurgical evaluation in focal epilepsy. Journal of Neurosurgery, 134,
1027-1036. https://doi.org/10.3171/2020.1.JNS192567
Jehi, L. (2018). The epileptogenic zone: Concept and definition. Epilepsy Currents,18(1), 12-16.
https://doi.org/10.5698/1535-7597.18.1.12 Luders, H.O., Engel, J. & Munari, C. (1993). General principles. In J.J. Engel (2nd Ed.), Surgical
Treatment of the Epilepsies (137-153). New York: Raven Press.
Maguire, M.J., Jackson, C.F., Marson, A.G. & Nevitt, S.J. (2020). Treatments for the prevention of
Sudden Unexpected Death in Epilepsy (SUDEP). Cochrane Database of Systematic Reviews,
Markoula, S., Chaudhary, U.J., Perani, S., De Ciantis, A., Yadee, T., Duncan, J.S., Diehl, B., McEvoy,
A.W. & Lemieux, L. (2018). The impact of mapping interictal discharges using EEG-fMRI on
the epilepsy presurgical clinical decision making process: A prospective study. Seizure:
European Journal of Epilepsy, 51, 30-37. https://doi.org/10.1016/j.seizure.2018.07.016
Mele, G., Cavaliere, C., Alfano, V., Orsini, M., Salvatore, M. & Aiello, M. (2019). Simultaneous EEG-
fMRI for functional neurological assessment. Frontiers in Neurology, 10(848).
Mohan, M., Keller, S., Nicolson, A., Biswas, S., Smith, D., Farah, J.O., Eldridge, P. & Wieshmann, U.
(2018). The long-term outcomes of epilepsy surgery. Public Library of Science One, 13(5).
Murta, T., Leite, M., Carmichael, D.W., FIgueiredo, P. & Lemieux, L. (2015). Electrophysiological
correlates of the BOLD signal for EEG-informed fMRI. Human Brain Mapping, 36(1), 391- 414. https://doi.org/10.1002/hbm.22623
Pittau, F., Grouiller, F., Spinelli, L, Seeck, M., Michel, C.M. & Vulliemoz, S. (2014). The role of
functional neuroimaging in pre-surgical epilepsy evaluation. Frontiers in Neurology, 5(31).
Rosenow, F & Luders, H. (2001). Presurgical evaluation of epilepsy. Brain, 124(9), 1683-1700.
Ryvlin, P. & Rheims, S. (2008). Epilepsy surgery: Eligibility criteria and presurgical evaluation.
Dialogues in Clinical Neuroscience, 10(1), 91-103. https://doi.org/10.31887/DCNS.2008.10.1/pryvlin
Sadjadi, S.M., Ebrahimzadeh, E., Shams, M., Seraji, M. & Soltanian-Zadeh, H. (2021). Localization
of epileptic foci based on simultaneous EEG-fMRI data. Frontiers in Neurology. 12(645594).
Shamshiri, E.A., Sheybani, L. & Vulliemoz, S. (2019). The role of EEG-fMRI in studying cognitive
network alterations in epilepsy. Frontiers in Neurology, 10(1033).
Sidhu, M.K., Stretton, J., Winston, G.P., McEvoy, A.W., Symms, M., Thompson, P.J., Koepp, M.J. &
Duncan, J.S. (2016). Memory network plasticity after temporal lobe resection: a longitudinal
functional imaging study. Brain, 139(2), 415-430. https://doi.org/10.1093/brain/awv365
Sidhu, M.K., Stretton, J., Winston, G.P., Symms, M., Thompson, P.J., Koepp, M.J., & Duncan. J.S.
(2015). Memory fMRI predicts verbal memory decline after anterior temporal lobe resection. Neurology, 84(15). 1512-1519. https://doi.org/10.1212/WNL.0000000000001461
Sinha, N., Dauwels, J., Kaiser, M., Cash, S.S., Westover, M.B., Wang, Y. & Taylor, P.N. (2017).
Predicting neurosurgical outcomes in focal epilepsy patients using computational modeling. Brain, 140(2), 319-332. https://doi.org/10.1093/brain/aww299
Sinha, N., Wang, Y., Moreira da Silva, N., Miserocchi, A., McEvoy, A.W., De Tisi, J., Vos, S.V., Winston,
G.P., Duncan, J.S., & Taylor, P.N. (2021). Structural brain network abnormalities and the
probability of seizure recurrence after epilepsy surgery. Neurology, 96.
Son, E & Kim, J. (2016). Spectrum of neurosurgeon’s role in epilepsy surgery. Biomedical Journal,
29, 177-182. https://doi.org/10.1016/j.bj.2016.01.009
Spencer, S. (2002). Neural networks in human epilepsy: Evidence of and implications for
treatment. Epilepsia, 43(3), 219-227. https://doi.org/10.1046/j.1528-1157.2002.26901.x
Szaflarski, J.P., Gloss, D., Binder, J.R., Gaillard, W.D., Golby, A.J., Holland, S.K., Ojemann, J., Spencer,
D.C., Swanson, S.J., French, J.A. & Theodore, W.H. (2017). Practice guideline summary: Use of fMRI in the presurgical evaluation of patients with epilepsy. Neurology, 88(4).
Szaflarski, J.P., Holland, S.K., Jacola, L.M., Lindsell, C., Privitera, M.D. & Szaflarski, M. (2008).
Comprehensive presurgical functional MRI language evaluation in adult patients with
epilepsy. Epilepsy & Behavior, 12(1), 74-83. https://doi.org/10.1016/j.yebeh.2007.07.015
Tavakol, S., Royer, J., Lowe, A.J., Bonilha, L., Tracy, J.I., Jackson, G.D., Duncan, J.S., Bernasconi, A.,
Bernasconi, N. & Bernhardt, B.C. (2019). Neuroimaging and connectomics of drug-resistant
epilepsy at multiple scales: From focal lesions to macroscale networks. Epilepsia, 60(4),
593-604. https://doi.org/10.1111/epi.14688
Taylor, P.N., Sinha, N., Wang, Y., Vos, S.B., De Tisi, J., Miserocchi, A., McEvoy, A.W., Winston, G.P., &
Duncan, J.S. (2018). The impact of epilepsy surgery on the structural connectome and its
relation to outcome. NeuroImage: Clinical, 18, 202-214.
Thornton, R., Vulliemoz, S., Rodionov, R., Carmichael, D.W., Chaudhary, U.J., Diehl, B., Laufs, H.,
Vollmar, C., McEvoy, A.W., Walker, M.C., Bartolomei, F., Guye, M., Chauvel, P., Duncan, J.S. &
Lemieux, L. (2011). Epileptic networks in focal cortical dysplasia revealed using
electroencephalography-functional magnetic resonance imaging. Annals of Neurology, 70(5), 822-837. https://doi.org/10.1002/ana.22535
Vakharia, V.N., Duncan, J.S., Witt, J., Elger, C.E., Staba, R. & Engel, J. (2018). Getting the best
outcomes from epilepsy surgery. Annals of Neurology, 86(4), 676-690.
Van Straaten, E.C.W. & Stam, C.J. (2013). Structure out of chaos: Functional brain network
analysis with EEG, MEG, and functional MRI. European Neuropsychoparmacology, 23(1), 7-
Wehner, T. & Luders, H. (2008). Role of neuroimaging in the presurgical evaluation of epilepsy.
Journal of Clinical Neurology, 4(1), 1-16. https://doi.org/10.3988/jcn.2008.4.1.1
Wellmer, J., Quesada, C.M., Rothe, L., Elger, C.E., Bien, C.G. & Urbach, H. (2013). Proposal for a
magnetic resonance imaging protocol for the detection of epileptogenic lesions at early
outpatient stages. Epilepsia, 54(11), 1977-1987. https://doi.org/10.1111/epi.12375
Wiebe, S., Blume, W.T., Girvin, J.P. & Euasziw, M. (2001). A randomized, controlled trial of surgery
for temporal-lobe epilepsy. The New England Journal of Medicine, 345(5), 311-318.
Zhang, J., Liu, W., Chen, H., Xia, H., Zhou, Z., Mei, S., Liu, Q. & Li, Y. (2014). Multimodal neuroimaging
in presurgical evaluation of drug-resistant epilepsy. NeuroImage: Clinical, 4, 35-44.
Zijlmans, M., Huiskamp, G., Hersevoort, M., Seppenwoolde, J.H., Van Huffelen, A.C. & Leijten, F.S.S.
(2007). EEG-fMRI in the preoperative work-up for epilepsy surgery. Brain, 130, 2343-2353.
Comentários